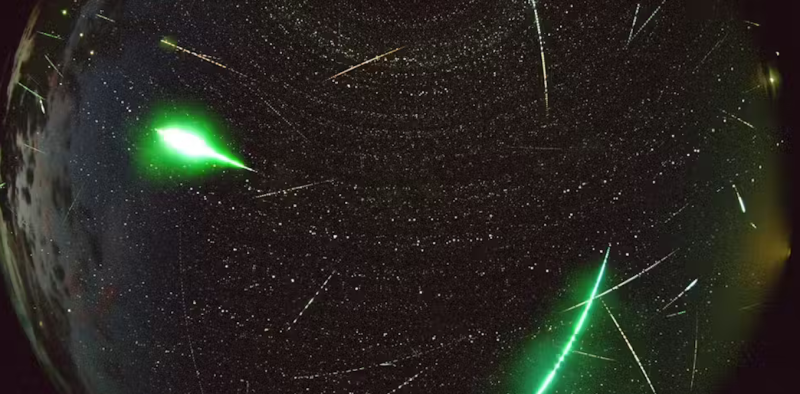
For decades, scientists have relied on meteorites – especially carbonaceous chondrites – to understand the early solar system. These ancient rocks, rich in water and organic compounds, are thought to have played a crucial role in delivering water to early Earth. However, a puzzling discrepancy exists: while observations of asteroids suggest that a large percentage should be carbonaceous, less than 4% of meteorites found on Earth fall into this category.
Recent sample-return missions like NASA’s OSIRIS-REx and JAXA’s Hayabusa2 have provided pristine samples from asteroids Bennu and Ryugu, respectively. These missions have given scientists a much clearer picture of the composition of these water-rich bodies, highlighting the differences between unaltered asteroid samples and the meteorites we find on Earth.
The challenge lies in the journey these rocks undertake. The Earth’s atmosphere acts as a filter, with weaker, more crumbly carbonaceous chondrites less likely to survive the fiery descent. But our research suggests that the atmospheric filter isn’t the only factor at play.
Utilizing global meteor and fireball observation networks like FRIPON and the Global Fireball Observatory, we tracked nearly 8,000 meteoroid impacts. By analyzing their trajectories and comparing them to the meteorites found on Earth, we uncovered a surprising finding: the Sun itself plays a significant role in reducing the number of carbonaceous chondrites reaching our planet.
The intense heat of the Sun, particularly during close orbital passes, causes thermal stress on asteroids. This stress creates cracks and fragments the weaker, water-rich carbonaceous material, effectively eliminating much of it before it even has a chance to reach Earth’s atmosphere. Those that do survive this solar baking tend to be more durable, and thus have a higher chance of surviving atmospheric entry.
This “survival bias” significantly impacts the meteorite population we observe on Earth, revealing that the scarcity of carbonaceous chondrites isn’t solely due to atmospheric filtering. Our findings highlight the need for further research, including improved telescopic detection of incoming objects, more refined atmospheric entry models, and advanced techniques for identifying meteoroid compositions based on meteor color.
By combining space-based sample return missions with ground-based observation networks, we are slowly piecing together a more complete understanding of the early solar system and the processes that shaped the composition of our planet. The mystery of the missing carbonaceous chondrites is a testament to the complexities of planetary science and the ongoing quest to unravel the secrets of our cosmic origins.